While it may be a surprise to others, just about any self-respecting reef aquarist knows that corals fight. Life on the reef can be tumultuous for a sessile, brainless colony of polyps. In addition to unforgiving environmental conditions and relentless predators, corals must contend with the existential threat posed by their own neighbors.
As coral colonies expand, space becomes an extremely limited commodity. To a colony, space is more than just figurative legroom, it very much so dictates their survival by facilitating the diffusion of gases, waste, and other vital nutrients, providing access to sunlight for photosynthesis, and allowing polyps to come into contact with prey items suspended in the water column. As a result, corals have evolved a myriad of different methods to defend themselves and their territory from encroachment.
The most common form of direct defense in corals comes from their cnidocytes. Like all other cnidarians, coral polyps possess specialized cells called cnidocytes that resemble bulb-like capsules covered by a small aperture that resembles a trapdoor. Within these cells reside specialized organelles called cnidae which vary in form and function. Cnidocytes essentially act like microscopic landmines, reacting to mechanosensory and chemosensory cues by everting and releasing their contents into the immediate surrounding area. Once everted, they may pierce, ensnare, or stick to surrounding structures.
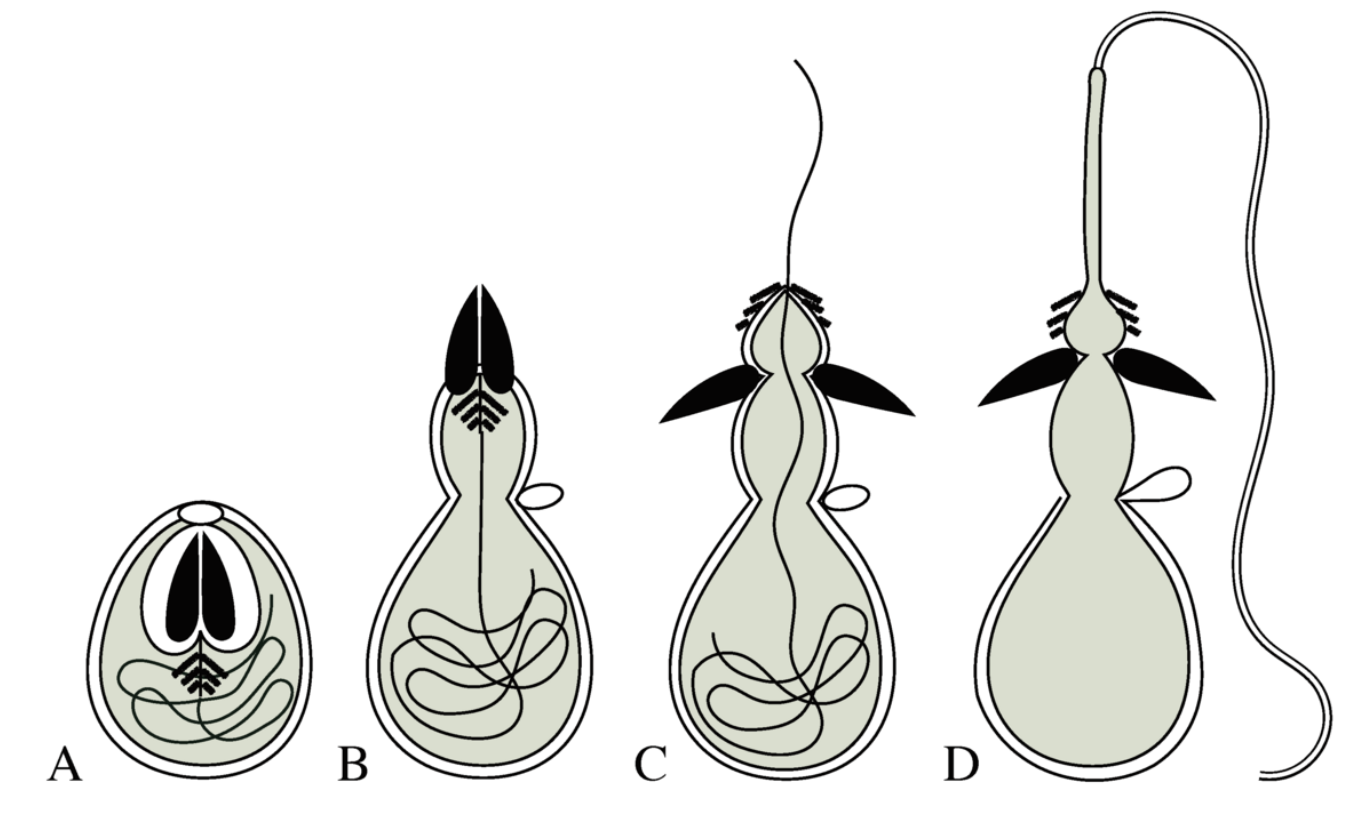
Cnidae are subdivided into three major categories. Nematocysts (~28 types), the most widespread and diverse cnidae, are responsible for the envenomation of prey and predators. Spirocysts (2 types) release a microscopic lasso-like thread that entangles surrounding structures. Finally, ptychocysts (found only in ceriantharian tube anemones), are used in the formation of protective structures. All cnidarians, whether an inconspicuous Discosoma sp. corallimorph to the most venomous box jelly have cnidae, often possessing multiple types. This collection of cnidae is collectively referred to as the animal’s “cnidom”. However, the cnidom of any one type of cnidarian does not necessarily possess all of the aforementioned types of cnidae.
Nematocysts and Envenomation
Many are probably familiar with the term “nematocyst”, one of the several types of cnidae that can be housed inside of a cnidocyte. Such cells that house nematocysts are appropriately termed “nematocytes”. Nematocysts often have a series of barbs or spines that allow them to puncture tissues when everted. Following puncture, neurotoxic venoms are delivered into the offending organism. Consequently, a nematocyte containing a nematocyst is often colloquially referred to as a “stinging cell”. These are the structures responsible for the painful injuries caused by cubozoans, jellies, and colonial hydrozoans like the Portuguese Man-o-War (Physalia physalis).
Nematocysts vary greatly in size and function, being further divided into many different classes (stenoteles, desmonemes, isorhizas, mastigophores, euryteles, etc) each of which have their own further categorical subdivisions. Despite this diversity, coral nematocysts generally fall into one of two categories – microbasic mastigophores and holotrichous isorhizas. These two types of nematocysts vary in their microscopic structure but serve the same function – which is to deliver venom.
Venoms are present in all species of cnidarians that possess nematocysts but little research has been devoted to those found in stony corals, which have been overshadowed in large part by jellies and sea anemones. Stony corals are known to possess proteinaceous toxins called small cysteine-rich peptides (SCRiPs) in their ectodermal tissues. Cnidarian SCRiPs are neurotoxic and affect the ion channels necessary for nerve function, causing near-immediate paralysis in small prey items for easier capture.
When it comes to predators, SCRiPs function the same way but are usually unable to overwhelm the nervous system of larger animals, instead eliciting a pain response. Depending on the type, cnidarian venoms can trigger nociceptors (pain receptors), disrupt neuronal functions, inactivate/activate ion channels, or attack muscle tissue by blocking the release of neurotransmitters – all of which are fairly good pathways for deterring any would-be predator
Large-scale studies show that stony corals likely have many other types of toxins in addition to SCRiPs, but no work has been done to identify and characterize these venoms at the time of writing. While their exact identities remain unknown, these toxins were shown to have hemolytic and nociceptive properties in toxicity assays with mice and crickets. Although these unidentified compounds were not found to be dangerous to humans, they have similar lytic effects on blood cells as toxins from hydrozoan “fire corals” (Millepora spp.).
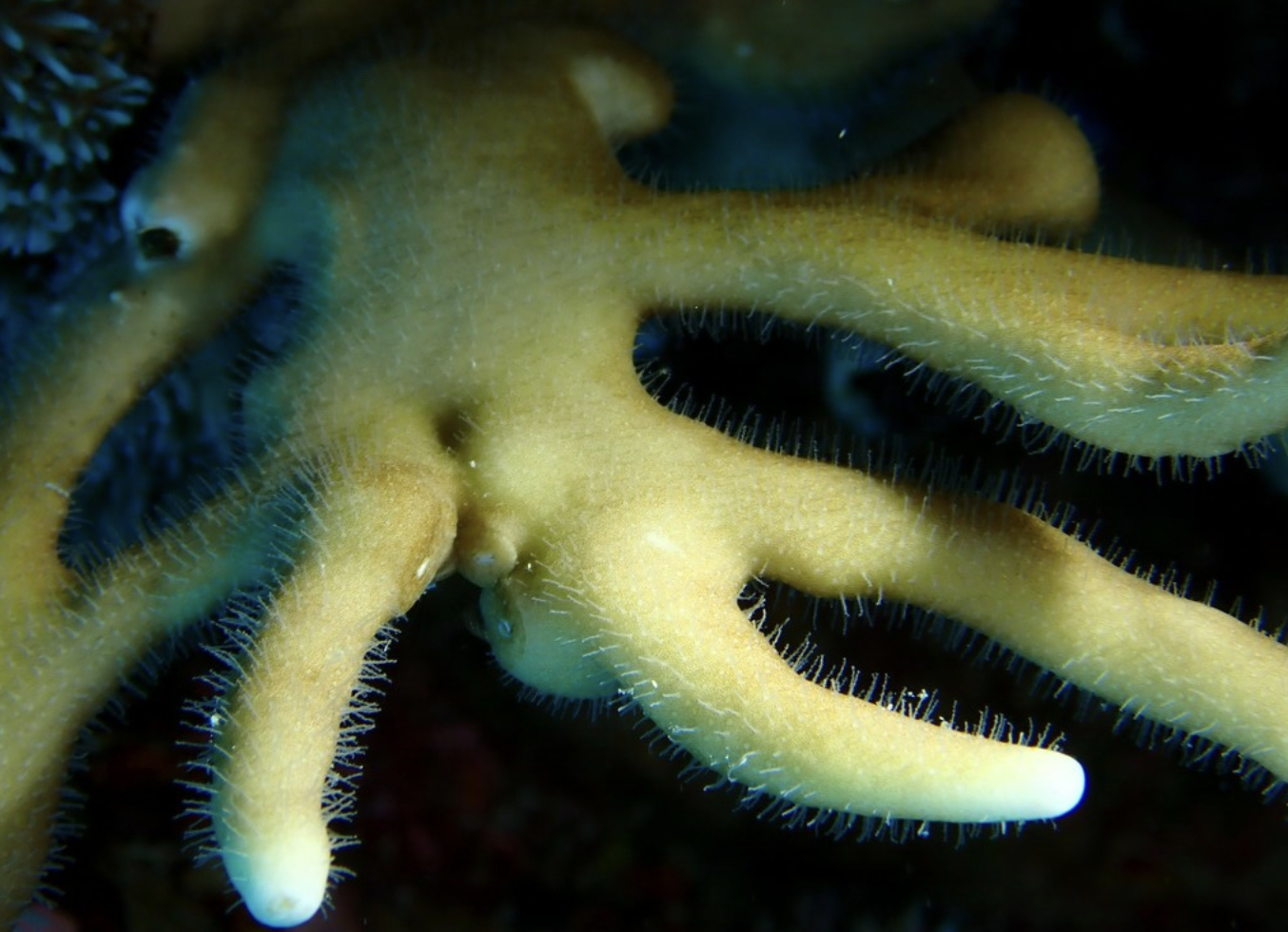
The pathways by which envenomation causes the tissue of a stung coral to recede, slough off, or necrotize are not entirely understood. The mechanism is likely similar to what is seen following bites/stings from other animals (snakes, spiders, scorpions, etc). In that case, a peer-to-peer coral sting may trigger a snowballing cascade of inflammatory responses independent of the venom’s effects, ultimately leading to localized cell death.
Given the aforementioned details on coral envenomation, the next logical thing to ask is why most people don’t feel the effects of a coral sting. The answer itself is quite simple. Coral nematocysts are incredibly small, often on the scale of dozens of micrometers (µm) long. These are not large enough to penetrate the protective outer layer of our skin, the epidermis. Our epidermal layer can be nearly half a millimeter thick at our fingers, thicker than most coral nematocysts (for reference, 1 mm = 1,000 µm).
However, other regions of our bodies with a thinner epidermis (eyes, underside of forearms, etc) can be penetrated by coral nematocysts. Aquarists who do report reactions from coral stings typically experience them on the undersides of their forearms and the back of their hands where the outer layer of skin is thinner.
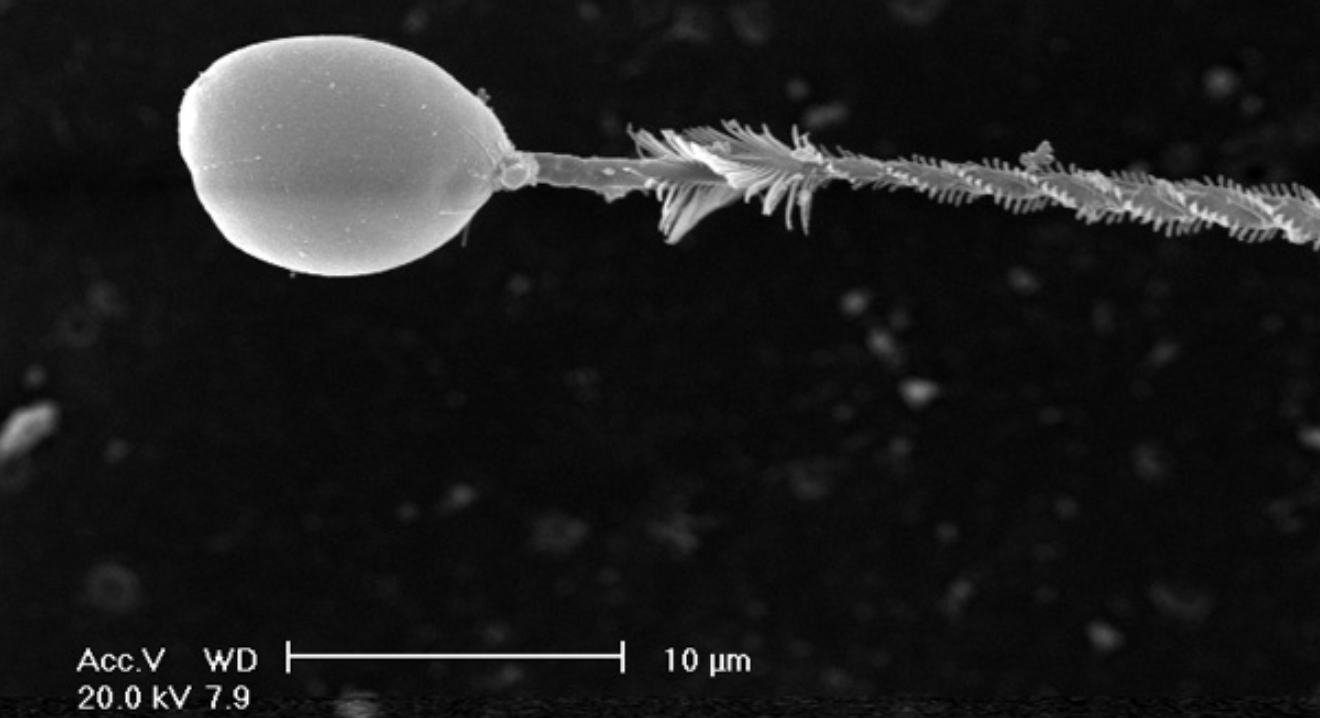
Sensitivity to coral envenomation varies greatly from person to person. Most aquarists tend to experience mild reactions to coral toxins (temporary erythema, swelling, itching), but more severe symptoms may occur in rarer instances. The worst I’ve found record of was a particularly bad case of cellulitis (which could have also been from a bacterial secondary infection), but that’s not to say that it couldn’t theoretically be worse. Although there are no known instances of major health-related events or fatalities from scleractinian or octocorallian stings, at least one individual has died following a sting from Condylactis gigantea, a species of sea anemone that is common in the reef aquarium trade.
Spirocysts and Ptychocysts
In addition to envenomating prey and predators alike, corals can also ensnare them. Spirocysts (released by spirocyte cells) are a trait unique to hexacorallian cnidarians (sea anemones, stony corals, and their closest relatives). These cnidae deploy identically to nematocysts, being fired in response to the right environmental stimuli, particularly chemosensory ones. However, instead of penetrating tissues when fired, spirocysts release a tendril into the surrounding water.
This tendril is covered in smaller microfibril tubules composed of a glue-like substance that solubilizes on contact with seawater, allowing the tendril to attach to almost any surface it touches. Microscopic drag forces cause these microfibrils to spin out like a web, maximizing their area of coverage. If you’ve ever felt a sticky sensation when touching a coral or anemone, or seen their tentacles stick to you, that was largely due to the action of spirocysts.
While there are dozens of different types of nematocysts, spirocysts are generally considered to be monomorphic, having just one major morphology shared across all taxa that possess them. Under a microscope, the capsules of these cnidae are slender and elongated with their filament stored in a characteristic corkscrew manner.
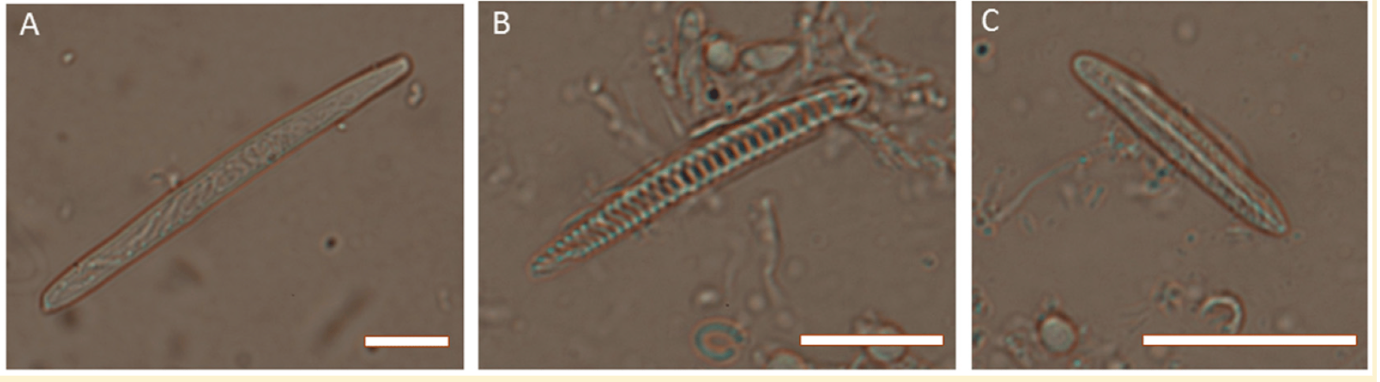
Not much is known about ptychocysts, the third class of cnidae. These were first described in 1977 and very little research has been conducted since then. Given that I’ve summarized a good deal of what’s currently known about them earlier (without getting too technical), I won’t babble any further.
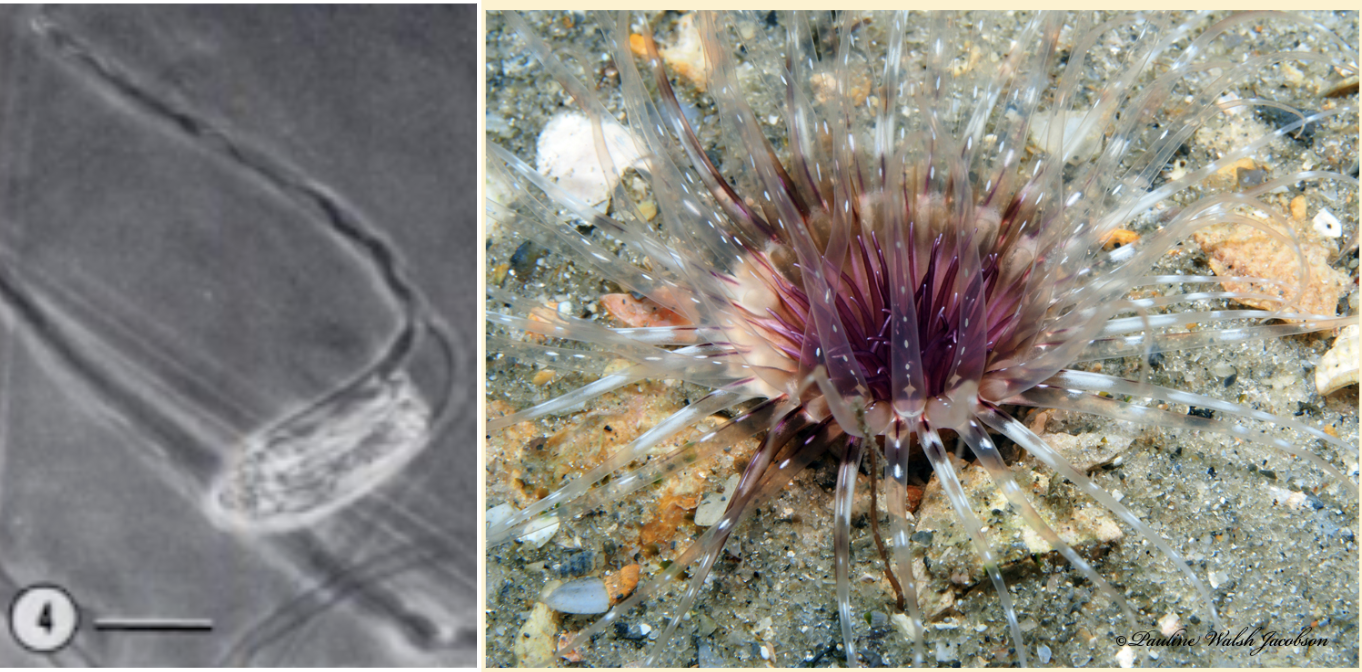
Batteries, Acrospheres, and Sweeper Tentacles
Cnidae are often organized along the tentacles of a coral polyp into “batteries” sharing a name with the military term for a group of artillery pieces. These structures are sometimes visible to the naked eye in some coral species, appearing as tiny white bumps on the sides of individual tentacles. A closer inspection reveals that cnidae batteries are actually an assemblage of different cells
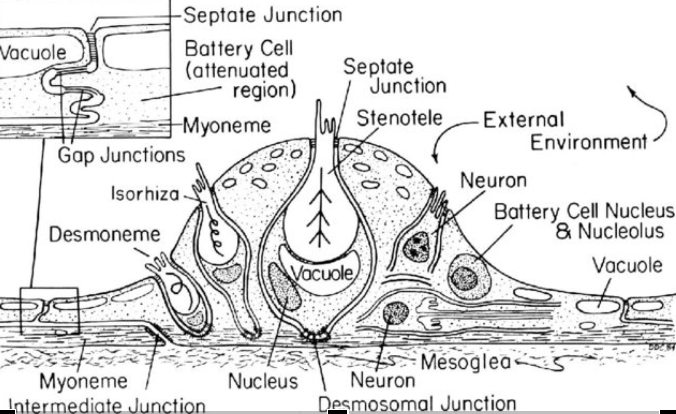
Many corals also possess a bulb-like structure at the tips of their tentacles called an “acrosphere”. These are essentially giant cnidocyte clusters positioned at the most distal point of the coral’s tentacles to maximize their effective “reach”. Both octocoral and stony coral species may possess acrospheres but they tend to be much more common in the latter, where they can take on rather elaborate shapes like those found in corals of the genus Fimbriaphyllia. The composition of cnidae within an acrosphere varies from species to species but most tend to have a near-equal mixture of nematocysts (microbasic b-mastigophores and/or holotrichous isorhizas) and spirocysts.
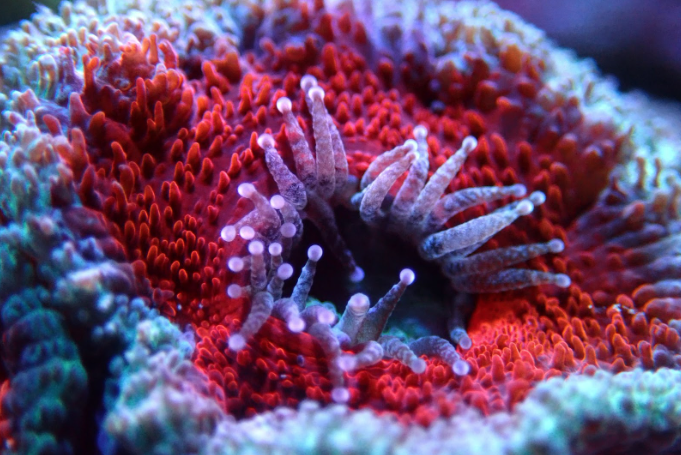
The range that corals can sting nearby organisms is typically quite limited, with normal feeding tentacles often extending only an inch or so beyond the oral disk of the polyp. However many coral species are known to produce elongated “sweeper” tentacles that can greatly expand their area of coverage. These whip-like tentacles can sometimes extend up to over a foot away from their origin point, giving them a massive advantage when it comes to spatial competition. Additionally, these tentacles pack a punch, with their cnidocyte composition being heavily skewed toward nematocysts, particularly microbasic b-mastigophgores.
Sweeper tentacles typically develop in response to direct contact with a neighboring coral’s tissues. These can form due to interactions both with different species but also with allogeneic tissues from different individuals of the same species. For example, two torch corals of different genetic backgrounds may both develop sweeper tentacles if they are close enough to each other, even though they are the same species (Euphyllia glabrescens). However, not all allogeneic combinations can develop sweeper tentacles and more research is needed to fully understand how and why they form. Sweeper tentacles are not permanent components of a coral’s anatomy, but instead form temporarily as needed and can take up to ~30 days to develop.
There is also some evidence that the development of sweeper tentacles is partially influenced by water flow. In a study involving the stony coral Galaxea fascicularis, sweeper tentacles formed under high-flow conditions. However, once the water flow stopped, these sweepers began to droop and retract. Over the course of a few hours, all of the colony’s sweeper tentacles had shrunken to the same size as the non-sweeper tentacles. If this phenomenon holds true with other coral taxa, it may be worthwhile to turn down flow within a reef tank after your lights turn off to minimize sweeper tentacle formation.
For the hobbyist, these sweeper tentacles simultaneously present a challenge when it comes to placing corals in the aquarium. Be sure to give corals (Galaxea, Fimbriaphyllia, Euphyllia, Plerogyra, Physogyra, etc) enough berthing space to prevent any stinging-induced fatalities of nearby colonies. Interestingly, while most aquarists seem to be familiar with the sweeper tentacles in stony corals, very few seem to be aware that many octocorals are capable of producing the same structures as well, albeit on a smaller scale.
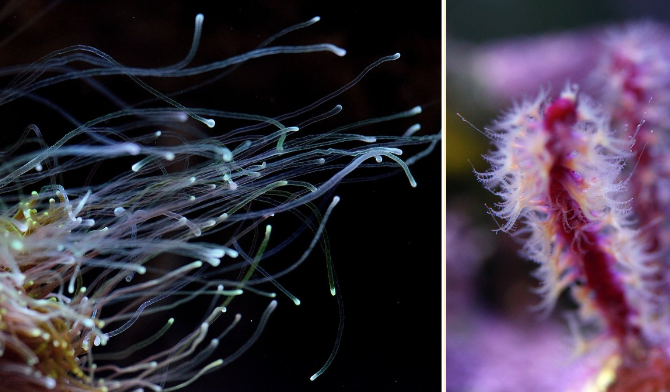
Mesenterial Filaments
Many corals also do battle by way of extracalicular digestion. Almost all species of stony corals possess squiggly, thread-like structures in their pharynx called mesenterial filaments. In most coral species, mesenterial filaments are usually a whitish color, but may be yellow (as in Duncanopsammia axifuga) or green (in some Rhodactis sp. corallimorphs). Under normal conditions, mesenterial filaments remain inside the coral’s pharynx, but under stress, these filaments may be used in interspecific competition.
Upon detection of chemical signatures indicative of a competitor species, certain corals may extrude their mesenterial filaments, expelling them from the opening in their pharynx (what aquarists typically refer to as a mouth) or through their body wall. When these mesenterial filaments are expelled onto a nearby organism, what ensues can result in the complete dissolution of the neighbor’s tissues – a process known as extracoelenteric digestion.
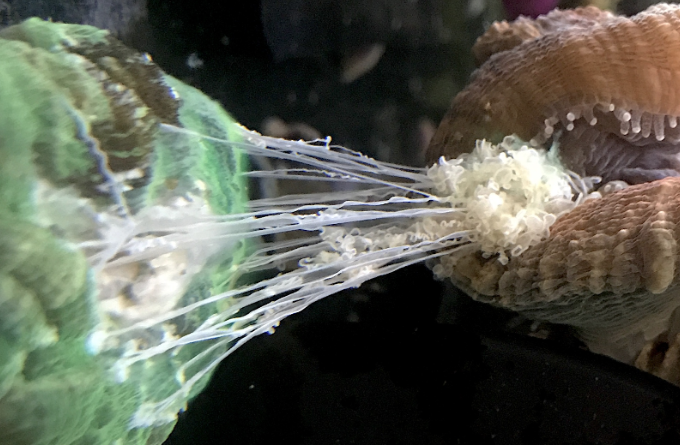
Very little research has been dedicated to classifying the digestive infrastructure of hermatypic stony corals, but one study did find that Stylophora possessed two different types of mesenterial filaments. One type was shorter and trilobed, having no stinging or secretory cells. The other type was much longer and had cytological graduation along their length, with alternations between secretory and stinging cells. These two types of mesenterial filaments work in conjunction with one another to digest organic matter, whether that may be inside the coral polyp’s pharynx or on top of a neighboring coral.
Chemical Warfare
Octocorals are known for possessing a myriad of toxic chemicals within their tissues. For the most part, these compounds serve anti-predatory functions. Many octocoral species possess chemicals that act as deterrents against predation by corallivorous fish, an effective primary dense via olfactory and/or gustatory cues. The tissues of some octocorals harbor even more potent toxins, ones that have been shown to have ichthyotoxic properties in laboratory studies.
Many of these ichthyotoxic compounds are secondary metabolites called diterpenes, a subclass of chemicals known as terpenoids which are often seen in the toxins of terrestrial plants. Octocorals that possess such chemicals usually rely on them almost exclusively for defense as at least one study has found a negative correlation between the presence of chemical deterrents and physical armament (spines, sclerites, etc). The presence of these ichthyodeterrent and ichthyotoxic chemicals provides some explanation as to why most corallivorous fishes tend to eschew many common octocoral species from their diets.
Terpenoids also serve a secondary function when it comes to spatial competition. Studies have shown that octocorals can release these toxins directly via contact with neighboring corals or indirectly into the water column. This process of antagonistic chemical warfare is known as allelopathy, a characteristic typically observed in plants, fungi, and bacteria. The effectiveness of allelopathic competition depends heavily on the species involved. When competing with other related octocorals, the results of allelopathy can be as minor as an immediate change in hydrostatic pressure within neighboring corals, causing them to bend away from the colony releasing chemicals into the water. Against, stony corals, allelopathy can result in slowed or inhibited growth, localized tissue necrosis, and even colony death.
While octocorallian soft corals are typically considered to be the sole culprits of chemical warfare in the home aquarium, at least one study has shown that stony corals are capable of this subversive form of spatial competition as well. Researchers in Brazil collected samples of secondary metabolites released from sun corals (Tubastraea tagusensis and T. coccinea) which are invasive in the region after simulating injury to the colonies. After analysis via gas chromatography-mass spectrometry (GC-MS), these chemicals were found to contain large amounts of fatty acids and sterols, indicating that they likely have antagonistic effects on other organisms. However, it remains unclear if stony corals like these Tubastrea spp. can release these substances on their own or only after being injured by predators.
Overgrowth
Coral warfare can also occur at a much slower pace, on a scale of weeks to months. While stinging and the expulsion of mesenterial filaments are often referred to as “overreach”, the gradual encroachment and smothering of surrounding corals is called “overgrowth”. With a finite amount of space available on any reef (wild or captive alike), corals eventually grow over one another, shading out smaller colonies and starving them of sunlight. In a reef aquarium setting, one might simply call this “suffering from success” and be left with some extra frags.
Conversely, on wild reefs, the overgrowth of some species (ex: foliose Montipora spp. and tabulate Acropora spp.) can spell certain death for smaller or less prolific corals. And like the tortoise in fables of old, slow and steady does indeed win the race. Over time, this strategy of physically shading out surrounding corals allows for a competitive edge on the reefscape This in part, is one of the reasons why the coral communities of more mature reefs tend to be less diverse and instead dominated by larger, horizontally-expansive species.
Conclusion
Corals are clearly much more capable combatants than most of us likely realized. In truth, this article barely scratches the surface when it comes to the complexity of the spatial competition we call “coral warfare”. Simply put, the mechanisms by which corals have evolved to defend themselves against competitors are as diverse as the corals that exhibit them.
Luckily, there are many simple yet effective ways to prevent and minimize the effects of coral warfare in the aquarium. By maintaining an adequate buffer zone between adjacent colonies (whether by positioning or fragging), aquarists can prevent most forms of overreach and overgrowth. Observing the tank at night or after feeding can provide insight into the reach of sweeper tentacles and allow for adjustments in flow or coral positioning to be made. Lastly, regularly replacing granular activated carbon (GAC) can be a cost-effective method of removing allelopathic compounds before they reach a toxic threshold in the water column.
Main image credit – Green Star Polyps: 1, Cyphastrea: 0 (Image Credit: Sean Ono)
References
Aceret, T. L., Sammarco, P. W., & Coll, J. C. (1995). Toxic effects of alcyonacean diterpenes on Scleractinian corals. Journal of Experimental Marine Biology and Ecology, 188(1), 63–78. https://doi.org/10.1016/0022-0981(94)00186-h
Ashwood, L. M., Norton, R. S., Undheim, E. A., Hurwood, D. A., & Prentis, P. J. (2020). Characterising Functional Venom Profiles of Anthozoans and Medusozoans within Their Ecological Context. Marine Drugs, 18(4), 202. https://doi.org/10.3390/md18040202
Barroso, R. A., Ramos, L., Moreno, H., & Antunes, A. (2024). Evolutionary Analysis of Cnidaria Small Cysteine-Rich Proteins (SCRiPs), an Enigmatic Neurotoxin Family from Stony Corals and Sea Anemones (Anthozoa: Hexacorallia). Toxins, 16(2), 75. https://doi.org/10.3390/toxins16020075
Chornesky, E. A. (1983). Induced development of sweeper tentacles on the reef coral Agaricia agaricites: A response to direct competition. The Biological Bulletin, 165(3), 569–581. https://doi.org/10.2307/1541466
Conklin, E. J., Bigger, C. H., & Mariscal, R. N. (1977). The Formation and Taxonomic Status of the Microbasic Q-mastigophore Nematocyst of Sea Anemones. The Biological Bulletin, 152(2), 159–168. https://doi.org/10.2307/1540556
Fautin, D. G. (2009). Structural diversity, systematics, and evolution of cnidae. Toxicon, 54(8), 1054–1064. https://doi.org/10.1016/j.toxicon.2009.02.024
Garcia, P. J., Schein, R. M. H., & Burnett, J. W. (1994). Fulminant Hepatic Failure from a Sea Anemone Sting. Annals of Internal Medicine, 120(8), 665. https://doi.org/10.7326/0003-4819-120-8-199404150-00007
Hawthorn, A., Berzins, I. K., Dennis, M. M., Kiupel, M., Newton, A. L., Peters, E. C., Reyes, V. A., & Work, T. M. (2023). An introduction to lesions and histology of Scleractinian corals. Veterinary Pathology, 60(5), 529–546. https://doi.org/10.1177/03009858231189289
Hidaka, M. (1985). Nematocyst discharge, histoincompatibility, and the formation of sweeper tentacles in the coral Galaxea fascicularis. The Biological Bulletin, 168(3), 350–358. https://doi.org/10.2307/1541517
Hidaka, M., & Yamazato, K. (1984). Intraspecific interactions in a scleractinian coral, Galaxea fascicularis: Induced formation of sweeper tentacles. Coral Reefs, 3(2), 77–85. https://doi.org/10.1007/bf00263757
Kass-Simon, G. (2002). The behavioral and developmental physiology of nematocysts. Canadian Journal of Zoology, 80(10), 1772–1794. https://doi.org/10.1139/z02-135
Kayal, M., & Adjeroud, M. (2022). The war of corals: patterns, drivers and implications of changing coral competitive performances across Reef Environments. Royal Society Open Science, 9(6). https://doi.org/10.1098/rsos.220003
Lages, B. G., Fleury, B. G., Rezende, C. M., Pinto, A. C., & Creed, J. C. (2010). Chemical composition and release in situ due to injury of the invasive coral Tubastraea (Cnidaria, Scleractinia). Brazilian Journal of Oceanography, 58(spe4), 47–56. https://doi.org/10.1590/s1679-87592010000800007
Mariscal, R. N., Conklin, E. J., & Bigger, C. H. (1977). The Ptychocyst, a Major New Category of Cnida Used in Tube Construction by a Cerianthid Anemone. The Biological Bulletin, 152(3), 392–405. https://doi.org/10.2307/1540427
Mariscal, R. N., McLean, R. B., & Hand, C. (1977). The form and function of cnidarian spirocysts. Cell And Tissue Research, 178(4). https://doi.org/10.1007/bf00219566
Na, S. Y., Lee, H. Y., Baek, J. O., Roh, J. Y., & Lee, J. R. (2008). A Case of Cellulitis Associated with Coral Injury. Annals of Dermatology, 20(4), 212. https://doi.org/10.5021/ad.2008.20.4.212
Raz-Bahat, M., Douek, J., Moiseeva, E., Peters, E. C., & Rinkevich, B. (2017). The digestive system of the stony coral stylophora pistillata. Cell and Tissue Research, 368(2), 311–323. https://doi.org/10.1007/s00441-016-2555-y
Paruntu C. P., Darwisito S., Angmalisang P. A., Rumengan A. P., 2022 The nematocysts of some Scleractinian coral species from the Sulawesi Sea, Indonesia. AACL Bioflux 15(5):2460-2470.
Sammarco, P. W., & Coll, J. C. (1992). Chemical adaptations in the Octocorallia: evolutionary considerations. Marine Ecology Progress Series, 88, 93–104. https://doi.org/10.3354/meps088093
Sammarco, P. W., La Barre, S., & Coll, J. C. (1987). Defensive strategies of soft corals (Coelenterata: Octocorallia) of the Great Barrier Reef. Oecologia, 74(1), 93–101. https://doi.org/10.1007/bf00377351
Schmidt, C. A., Daly, N. L., & Wilson, D. T. (2019). Coral Venom Toxins. Frontiers in Ecology and Evolution, 7. https://doi.org/10.3389/fevo.2019.00320
Sebens, K. P., & Miles, J. S. (1988). Sweeper tentacles in a gorgonian octocoral: Morphological modifications for interference competition. The Biological Bulletin, 175(3), 378–387. https://doi.org/10.2307/1541729
Thorington, G. U., & Hessinger, D. A. (1990). Control of Cnida discharge: III. Spirocysts are Regulated by Three Classes of Chemoreceptors. The Biological Bulletin, 178(1), 74–83. https://doi.org/10.2307/1541539