Given their common name of soft corals, one would likely assume that octocorals are well… soft. However, as is common in biology, there are always exceptions to rules. Within the Octocorallia, there are a few species that form aragonite-based skeletons, most of which belong to the family Helioporidae.
Helioporids are enigmatic octocorals from the order Scleralcyonacea with uncertain evolutionary origin. The earliest records of Heliopora fossils suggest the genus has been around since at least the Cretaceous and were once widely spread throughout the world’s warm oceans. At one point they were also the primary contributors to reef formation, prior to the evolutionary radiation of modern scleractinian stony corals. These “living fossils” have endured at least two mass extinction events and incredible environmental dynamism, yet have hardly changed. The late Japanese coral biologist Motoki Eguchi said “Notwithstanding its great antiquity, it differs very little from the living Heliopora coerulea”, in reference to the oldest representative of the genus Heliopora, an extinct species called H. japonica. The family Helioporidae contains a grand total of three extant species between its two genera, Heliopora and Nanipora, the latter of which is remarkable in its own right.
Like all other octocorals, Heliopora exhibit polyps with octamerous symmetry The largest of these polyps, called autozooids, are only a few millimeters across when fully extended. Each of the eight tentacles on an autozooid polyp is flanked by minute pinnules, barely visible even with a good macro lens. Autozooids are the colony’s primary method for prey capture and defense against predators. However, the nematocysts responsible for stinging prey or predators are quite tiny, reaching a maximum capsule length of 9 ?m (1 millimeter is equivalent to 1,000 micrometers), some 2-3 times smaller than the typical nematocysts found in stony corals.
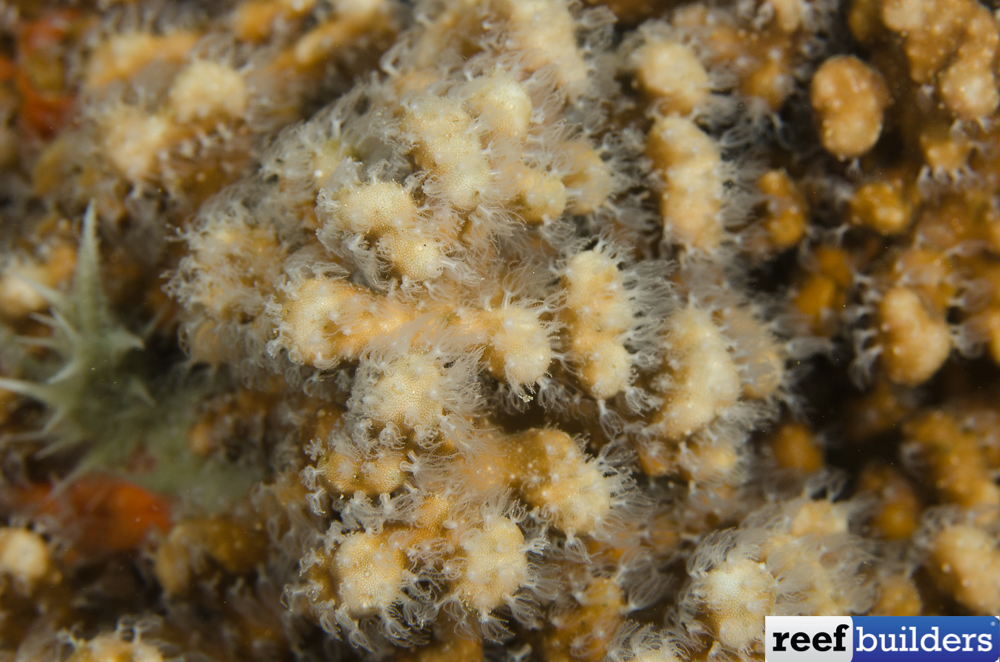
While the structural integrity of most octocorals comes from a disconnected framework of calcite sclerites, Heliopora are known for depositing a solid skeleton of fibrocrystalline aragonite, similar to that of scleractinian stony corals. However, according to at least one study, Heliopora have lower rates of calcification than branching, corymbose, digitate, and massive stony coral species, likely due to the dominance of thermotolerant Symbiodinium sp. dinoflagellates within its holobiont. Consequently, Heliopora have an optimal growth temperature that approaches and may even exceed the upper thermal limit at which most stony corals would bleach. In the wild, this manifests with Heliopora having an extremely shallow depth range and an exclusively equatorial distribution. Some studies have even suggested that Heliopora might benefit from rising ocean temperatures but these claims warrant further investigation. Nonetheless, Heliopora are considered hermatypic (i.e. “reef-building”) and make significant contributions to the overall deposition of calcareous material on coral reefs.
On wild reefs, Heliopora coerulea is highly polymorphic and takes on a wide range of growth habits. Colonies may be digitate, columnar, vertically plating, or something in between. Heliopora occur in rather shallow waters from intertidal zones to depths of around 10 meters where they can be the dominant coral species. In many instances throughout their range, Heliopora colonies can form expansive thickets that span dozens or even hundreds of meters in breadth. Large colonies that form vertical plates can sometimes be confused for “fire corals” (Millepora spp.) which are reef-building hydroids known for their injurious stings. Ironically, Heliopora coerulea was originally described as Millepora coerulea back in 1766.
These colonies often harbor the endolithic spionid worm Dipolydora armata which is a facultative commensal that forms tubes in various calcareous substrates including living coral skeletons. These worms are sometimes thought to be parasitic since they physically bore into their coral host, but given their diminutive size, they likely cause little if any damage to the colony. Through personal correspondence with a marine annelid researcher, I’ve learned that spionid worms may actually be beneficial to their coral hosts since they selectively feed on organic particles and can help keep corals free from settled detritus, dead tissue, and exudate.
Perhaps the most distinctive aspect of Heliopora skeletons is their ubiquitous blue pigmentation, the origin of their common name “blue coral”. Outwardly, Heliopora are brown on account of their copious dinoflagellate endosymbionts so the name “blue coral” may seem counterintuitive at first. However, broken or bleached colonies reveal their bright blue interior. This color is the result of the accumulation of a pigment called Biliverdin IX?. Biliverdin is produced by the decomposition of heme (a ring-shaped iron molecule) in heat-shock proteins by the enzyme heme oxygenase during oxidative stress. The termination of this metabolic pathway typically ends with the conversion of biliverdin into bilirubin by an enzyme called biliverdin reductase. Metatranscriptonomics have revealed that Heliopora do not contain biliverdin reductase enzyme, meaning that while they can synthesize biliverdin they cannot metabolize it, causing the pigment to build up in their skeletons and produce that vivid blue hue.
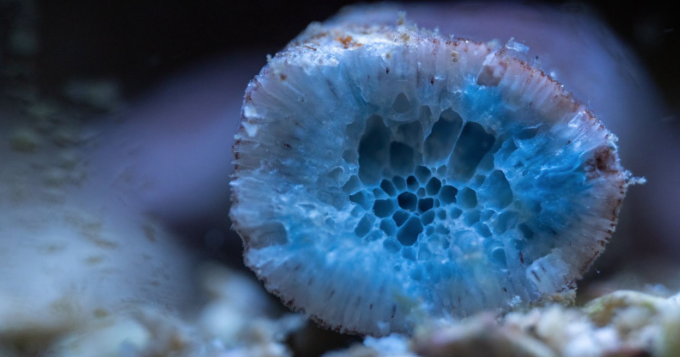
Heliopora coerulea is also notable for being well-documented as a surface-brooding species. H. coerulea is gonochoric (having separate male and female colonies) and follows an annual gametogenic cycle with lunar or semi-lunar periodicity, meaning that like many other corals, they spawn just once per year during either the full or new moon. However, unlike many corals, rather than broadcast spawning sperm and eggs into the water column, male H. coerulea release sperm into the water which is subsequently captured by female colonies where it fertilizes their oocytes internally. The resulting larvae are brooded inside the mother colony’s polyps for a period lasting between 6 and 14 days. During this time, these planula-bearing autozooid polyps will expand greatly in size, appearing inflated and giving parts of the coral a pillowy appearance. One study suggests that the distension of brooding autozooids is an adaptation that elevates planulae above detritus that accumulates in the colony’s recesses, giving them a potential survivorship advantage in sediment-impacted reefs. Another study found that the planulae of Heliopora coerulea were almost 4 times larger than those of broadcast spawning corals like Pocillopora damicornis and had an associated higher rate of recruitment. The large planulae of Heliopora seem to have limited mobility (on account of decreased buoyancy from low lipid content), which results in smaller areas of distribution, but this proximity to the benthos leads to increased recruitment success.
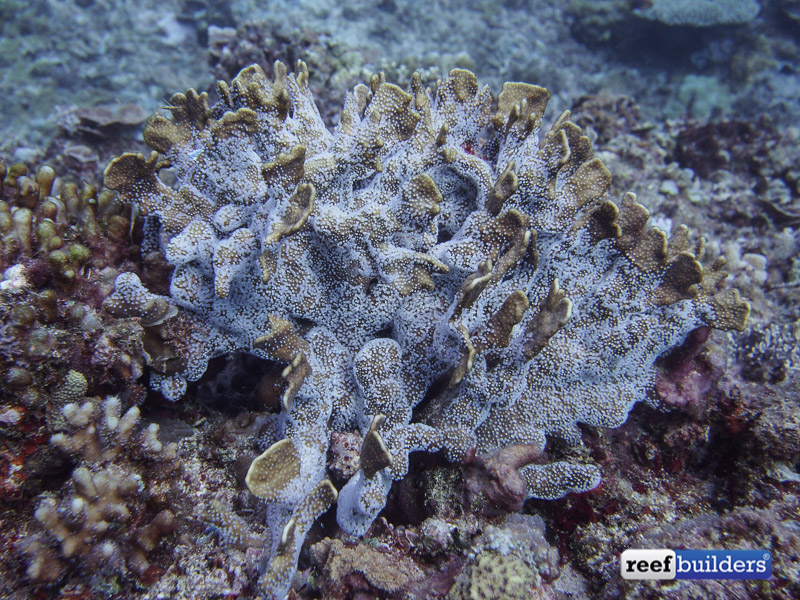
For many years, H. coerulea was considered to be the only extant species within the genus Heliopora. However, in 2018, a new species called H. hiberniana was described with a combination of morphological, genomic, and reproductive evidence. This new species was known from and named for the Hibernia Reef of Western Australia but has more recently been discovered in the Maldives and Indonesia. Despite occurring sympatrically, Heliopora hiberniana is morphologically, genetically, and reproductively distinct from its congener H. coerulea.
At a glance, it appears to be much more delicate, having thinner branches that grow in a spindly or digitate colony. Under the microscope, one would find that the microstructure is also markedly different. Their autopores (basically a convergently-evolved corallite in skeleton-building octocorals) are smaller and much more numerous than those of H. coerulea. Finally, their echinulations are much more elaborate as well. These microscopic structures are multi-pronged (quite frankly they remind me of human molars), whereas those in H. coerulea are simple unipoint spines. Oddly enough, H. hiberniana also seems to never host the endolithic spionid worms that frequent H. coerulea.
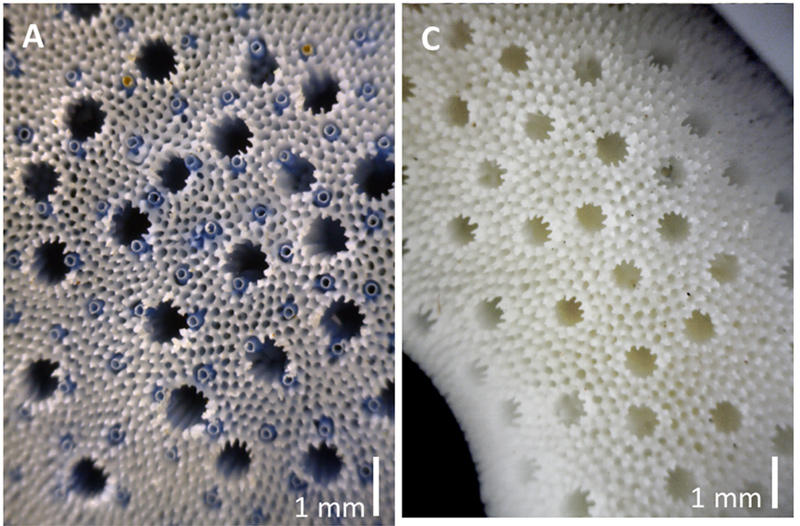
Another striking difference is the massive departure from the trait behind its namesake of “blue coral”. Unlike H. coerulea, H. hiberniana does not develop a blue color in its skeleton and instead, the interior takes on the white color of the fibrous aragonite that it is composed of. Heliopora hiberniana likely possesses the enzymes necessary to metabolize biliverdin, but another metatranscriptome study is needed for this species before any assumptions can be made.
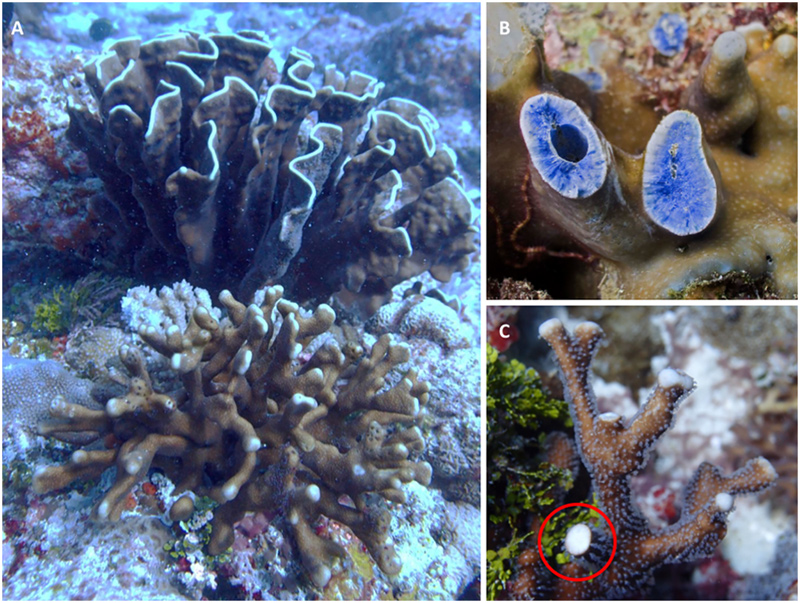
Given its novel nature, little is known about the reproductive biology of Heliopora hiberniana. However, preliminary inspections show that mature colonies harbored oocytes within their gastric cavity rather than brooding planulae in their polyps like H. coerulea. This implies that H. hiberniana is not a surface brooder like its congener. In theory, this makes sense since the thinner branches provide much less protection from biotic and abiotic disturbances. Again, further study on this species is needed to better understand its biology.
In recent years, phylogeographic work has found data that suggests there are likely more Heliopora species than we currently recognize. Genetics suggest there are three major clades within the Heliopora genus: the H. coerulea group, the H. hiberniana group, and an undescribed Heliopora group. Although the authors of the study do not formally describe the new Heliopora group or any other subgroups, they do note that there is substantial genetic diversity within the three aforementioned clades. If each of the eight genetically isolated lineages across the three species groups turns out to be distinct species, we could see the genus Heliopora quadruple in size in the coming years. For now, we’ll have to wait for the researchers to conduct more work before any exciting announcements can be made.
While Heliopora are becoming increasingly uncommon in the modern reef aquarium hobby, I hope that after reading this, you are inspired to give this utterly fascinating octocoral a try.
Literature Cited
Babcock, R. (1990). Reproduction and development of the Blue Coral Heliopora coerulea (Alcyonaria: Coenothecalia). Marine Biology, 104(3), 475–481. https://doi.org/10.1007/bf01314352
Colgan, M. W. (1984). The cretaceous coral Heliopora (Octocorallia, Coenothecalia)—a common Indo-Pacific Reef Builder. Casebooks in Earth Sciences, 266–271. https://doi.org/10.1007/978-1-4613-8271-3_33
Courtney, T. A., Guest, J. R., Edwards, A. J., & Dizon, R. M. (2021). Linear extension, skeletal density, and calcification rates of the Blue Coral Heliopora coerulea. Coral Reefs, 40(5), 1631–1635. https://doi.org/10.1007/s00338-021-02137-3
Hongo, Y., Yasuda, N., & NagaI, S. (2017). Identification of Genes for Synthesis of the Blue Pigment, Biliverdin IX?, in the Blue Coral Heliopora coerulea. The Biological Bulletin, 232(2), 71–81. https://doi.org/10.1086/692661
Harii, S., Kayanne H., Takigawa H., Hayashibara T., & Yamanoto M. (2002). Larval survivorship, competency periods and settlement of two brooding corals, Heliopora coerulea and Pocillopora damicornis. Marine Biology, 141(1), 39–46. https://doi.org/10.1007/s00227-002-0812-y
Toh, T. C., Soon Lionel Ng, C., Ben Toh, K., Afiq-Rosli, L., Taira, D., Loke, H., & Ming Chou, L. (2015). Mass brooding of the blue octocoral, Heliopora coerulea on a sedimented equatorial reef. Marine and Freshwater Behaviour and Physiology, 49(1), 69–74. https://doi.org/10.1080/10236244.2015.1112959
Zann, L. P., & Bolton, L. (1985). The distribution, abundance and ecology of the Blue Coral Heliopora coerulea (Pallas) in the Pacific. Coral Reefs, 4(2), 125–134. https://doi.org/10.1007/bf00300871
About the Author
Sean Ono has been keeping corals for the better part of a decade, both at home and as a former volunteer aquarist at one of the largest public aquariums in the United States. He has a particular affinity for anthozoan cnidarians (including corals) but is passionate about echinoderms, marine worms, macroalgae, and more. Despite his familiarity with reef-associated organisms, his previous academic research is more elasmobranch-centric, aiming to quantify the biomechanical and behavioral aspects of stingray tail strikes. Having recently graduated with a Bachelor of Science in Marine Biology, Sean hopes to pursue a Ph.D. in ecology and evolution, focusing on the intersection of biomechanics, sensory capabilities, and comparative morphologies of marine taxa.
(adsbygoogle = window.adsbygoogle || []).push({});