In this installment of the microbiology series, I will provide a more technical explanation of the coral immune response.
Introduction to Immunology
Immunology is the study of the immune system. There are two broad categories of immune responses – innate and adaptive. Innate immunity is the automated response of an organism to infection – it can deal with any external invader and is ever-present. Adaptive immunity is where the big guns are. In humans, these are the humoral immune systems involving B and T cells as well as antibodies. Adaptive immunity involves specialized cells that can catalog external invaders and then mount a formulated response against them – typically calling in the help of the innate immune system. The cataloged species are then stored and saved, which allows for a much faster response if that pathogen ever enters the body again.
This concept is the crux of immunity and vaccination to certain diseases – if you get the flu and get infected with that same strain again, you will be much less ill the second time. While most people are familiar with the basic concepts surrounding how the human or even mammalian immune systems function, much of this goes out the window when it comes to coral. Before I dive into the nuances of the coral immune system, I will introduce you to a few of the specialized cells we will discuss more in-depth below and give a brief overview of several immunological pathways.
Players In The Game
Before diving into the specific players, it’s essential to understand the game’s rules. A good analogy is to imagine the immune system like a McDonalds. There’s a litany of specialized immune cells that are very skilled at what they do in this instance: it’s eliminating foreign invaders (you can liken these to the cooks who can seemingly manifest a mouthwatering quarter pounder, 10-piece nugget, and extra-large fry from thin air).
While these immune cells are excellent at defending you, they can’t operate unless they receive an order – that’s where signaling molecules come in. Much like the person (or now AI) taking your order at the drive-through and delivering it to the cooks, once pathogens enter the body and are recognized, detection proteins activate various signaling pathways. These signals then activate the immune cells to find and eliminate the pathogen.
You pull up in the drive-through and place your order. The cooks then receive a message and prepare your meal. A pathogen enters a coral, is recognized, and activates signaling molecules, which turn on specialized immune cells that eliminate it—a happy customer and coral. The primary pathway to remember here is recognition -> signaling -> elimination.
Recognition
Much like a sensor detecting you’re at the drive-through, many receptors exist on cells that can detect the presence of pathogens once they enter the body. A good way to imagine this interaction is to think of pathogens possessing specific regions with Velcro. Immune detection cells also have Velcro on some parts of them – thus, if an immune cell and pathogen were to bump into each other, they would momentarily “stick” together through the two pieces of Velcro meeting. This binding tells the immune cell that trouble is up, which lets it sound an alarm and activates signals to turn on elimination cells recruited to eradicate the pathogen. Here are a few recognition structures and molecules to be familiar with.
– TLR = Toll-like receptors – located on the external membrane of cells where they recognize patterns found on pathogens.
– PRR = a receptor belonging to the host that can bind to and recognize DAMPs and MAMPs.
– Lectin = a protein that binds to carbohydrates – in this instance, ones specific to carbohydrates found on a pathogen.
– DAMPs = Danger-associated molecular patterns – specific compounds or structures produced by stressed or damaged cells – these can include proteins, reactive molecules, or compounds such as uric acid.
– MAMPs = Microbe-associated molecular patterns – specific features a microbe possesses that allows it to be identified – proteins or receptor sites on their external membrane.
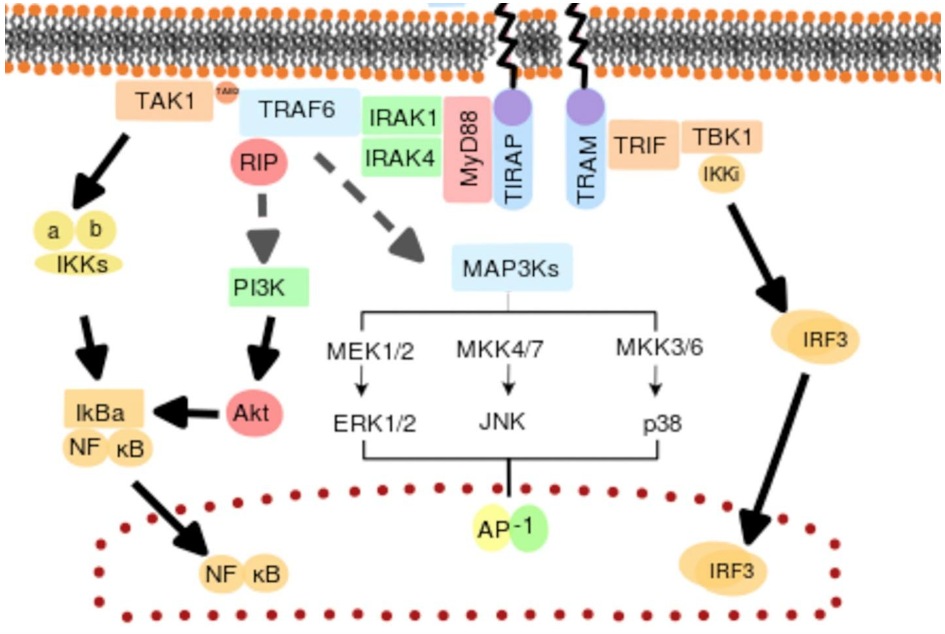
Signaling
After recognizing a pathogen, signaling cells produce or recruit signaling molecules that deliver the message to elimination cells. While various types of signaling can occur in corals, we will focus on one primary mechanism for the sake of article length.
– Cytokine = small proteins that can dissolve and quickly travel around a body. They then bind to immune cells, which can activate them, alerting them to an infection.
Elimination
There are a variety of immune cells the innate immune system can utilize to deal with pathogens – each has advantages and disadvantages, and often these are deployed in combination. When an organism is healthy, these cells lay dormant, waiting to be activated by cytokine signaling.
– Macrophages = large cells that engulf pathogens or infected cells – an example of these in the human body are white blood cells (the main image of this article shows one eating a bacterium under an electron microscope).
– Complement = a complex pathway consisting of particles that can act like cytokines and direct macrophages to consume damaged or foreign cells. It also includes constructing and deploying the membrane attack complex (MAC), which can bind to undesirable cells and eliminate them by poking dozens of holes in them.
– Natural killer cells = janitors of the immune system – they recognize dead or defective cells and induce apoptosis in them (programmed cell death).
– Interferons = specialized cytokines designed to eliminate viruses – some bind to double-stranded RNA, a typical intermediate viruses use to replicate, while other classes can mark virus-infected cells for elimination or activate more comprehensive viral responses.
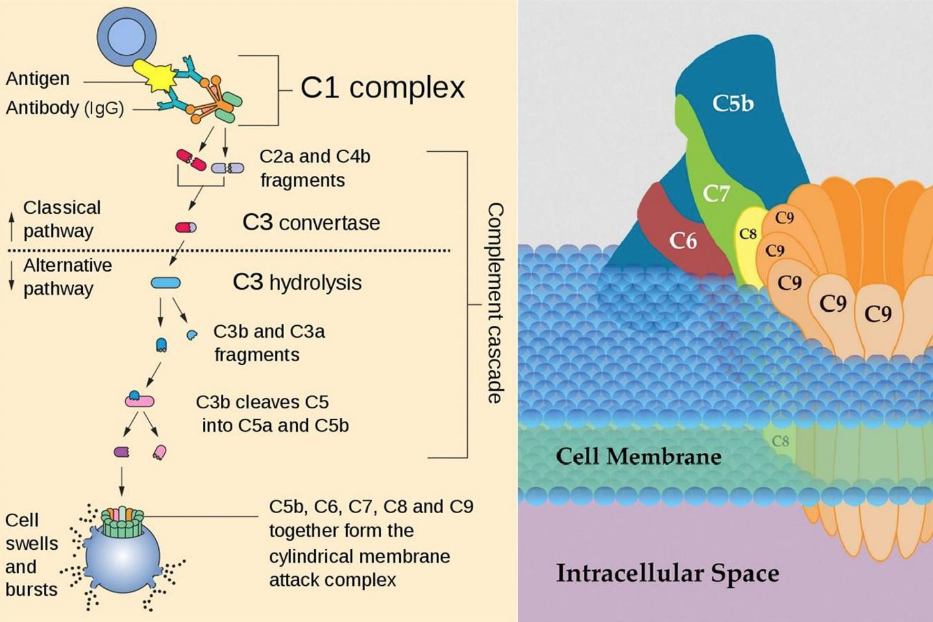
Li, D. (2022, October 24). Complement. Complement – Immunology – Medbullets Step 1.
https://step1.medbullets.com/immunology/105052/complement
Self vs Non-Self
Now that we have discussed the innate immune system and its components at a broad level, we will analyze specific theories of immune response and how they pertain to corals. Self vs. non-self is the typical style of immune response that invertebrates utilize. If a pathogen enters the body, the host recognizes it as foreign, and then the pathway recognition -> signal -> elimination occurs. In nearly all infections observed in corals, this pathway is active. There’s just one problem – the holobiont is composed entirely of non-self.
For years, researchers have asked how a coral can simultaneously detect and eliminate pathogenic microbes and allow millions of beneficial foreign microbes to live in and on them. Data has illustrated that the innate immune system recognizes beneficial microbes through the exact mechanisms as pathogenic ones, but for some reason, they don’t trigger a signal that leads to elimination – how can corals delineate between friend and foe if both are non-self?
Danger Theory
Another pathway observed in corals is the danger system. Typically, this is a response to intercellular stress. Dying or damaged cells produce DAMPs, which are then recognized and trigger signaling, activating both the immune and repair systems. This systemic response is termed an integrated stress response (ISR). Corals initiate an immune response when cellular damage occurs for two primary reasons. First, cellular damage may be biotic in origin, caused by a pathogen, or second, abiotic, which can leave an open wound for pathogens to enter (such as someone using a bandsaw to frag their coral).
This mechanism usually takes a backseat to many organisms’ primary self/non-self-system, but new models suggest it may be the missing piece of the coral immune puzzle.
The Damage Threshold Hypothesis
Researchers have developed a new theory for how the coral immune system operates that can effectively explain the issue of the holobiont. This idea, known as the damage threshold hypothesis, combines self/non-self-recognition with the danger model, positing a hybrid mechanism where a coral must recognize a foreign invader and a danger signal to activate an immune response.
This model makes sense as recognition proteins would undoubtedly identify the beneficial microbes of the holobiont as non-self. Because their presence does not cause cellular damage, no coral cells will produce DAMPs; thus, the immune system does not eliminate the symbionts. Conversely, cellular death will occur if a pathogenic microbe invades a coral, leading to non-self-recognition and danger signal production.
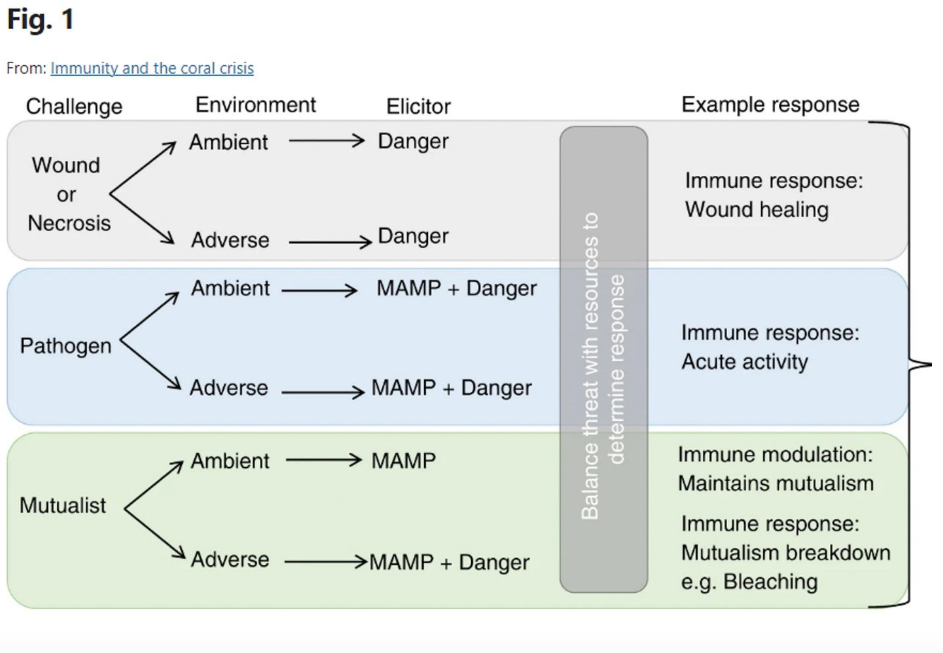
Palmer, C. V. (2018, July 9). Immunity and the coral crisis. Nature News.
https://www.nature.com/articles/s42003-018-0097-4#Sec10
This model also explains one of the most puzzling phenomena observed in corals – bleaching. Intuitively, it makes no sense that when stressed, a coral would eliminate a significant source of its energy. Doing so almost guarantees the coral’s death since few corals recover from bleaching events. Instead of this being an intentional act, the damage threshold model explains that zooxanthella themselves will produce DAMPs during times of abiotic or biotic stress, even if the coral is not as stressed. Thus, this satisfies the two checkpoints the coral immune system must pass to mount a response.
The zooxanthella are then swiftly eliminated because they are recognized as non-self and provide a localized source of DAMP’s. Bleaching is the coral’s immune response on full display – a visual cue of how effectively it can protect itself.
Putting It All Together
Let’s create a hypothetical scenario to craft a complete picture of how the coral immune response functions with the damage threshold hypothesis. I’m a hobbyist who saved up and purchased an RRC rainbow splice. After months of cooking, she’s a beaut – I’ve grown my frag into a colony. I decided to make a backup frag to put in one of my other systems, so I took some bone cutters and nipped off a branch. I then utilized the tried-and-true glue-epoxy-glue method and even dipped it in iodine. I put it back into my display tank on a magnetic rack.
After two weeks, to my amazement, it survived fragging. I then transferred it into another system. A week later, I noticed that my frag was getting pale – I checked the PAR and all significant elements and realized it was identical to my primary system, but my pH was slightly lower. I sent in Aquabiomics and ICP and ruled out any substantial pathogens or redundancies in trace elements. After another week, my frag sadly died. What happened?
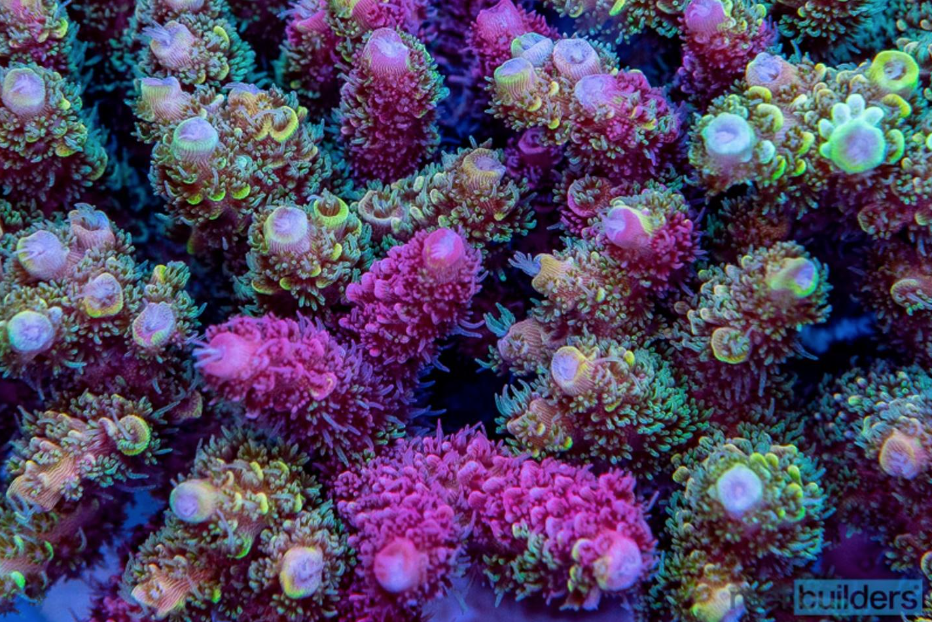
In this hypothetical scenario, let’s assume that the stress was entirely abiotic and that a pathogen was not involved. The acro frag most likely bleached slowly due to the shift in pH when it was transferred to another system. The zooxanthella, microbial member of the holobiont, or the coral itself may have been stressed enough to where they produced danger signals, and then the immune system slowly purged the zooxanthellae from its system.
While we often think of bleaching as a fast phenomenon, the damage threshold hypothesis posits that auto-immune bleaching would occur at a rate proportional to the damage signal – thus, the more stressed the organism, the faster it will bleach. If the Acropora only went through a pH swing and no other swings, this could lead to a slow death instead of a rapid one.
This scenario is quite unrealistic as, in reality, after fragging and transfer, our corals deal with both biotic and abiotic stress, which makes the issue of preventing coral death much more complicated to both diagnose and treat than simply “it was a pH swing” or “it was vibrio.” One of the issues lies in the fact that the current zeitgeist of the community is to rush to the assumption that their coral has contracted unknown pathogen X, and that’s the root cause of their issues when data suggest pathogenic events are tied to abiotic stressors.
Additionally, there may be purely abiotic events from improper chemistry or due diligence, resulting in an immunological tactic where the coral essentially “shoots itself in the foot.” Auto-immune bleaching (AMB) may explain some of the cases of STN and slow bleaching observed in the hobby that few remedies tend to address.
Life and reefing are rarely black and white – the truth is often grey. If anything, I hope this explanation and hypothetical scenario help you consider that grey area by highlighting that abiotic stress is at the table next to pathogenesis when it comes to unknown or sudden coral death, especially when considering that the damage threshold of freshly imported corals is likely significantly lower than that of aquacultured ones.